Superfluidity of ultracold fermions
Superfluidity of ultracold fermions
Ultracold fermions are one of the most versatile systems known to exist for research on superfluid matter. The superfluid property is stabilized by interactions and ultracold fermions provide the unique possibility to tune these interactions at will, with the help of a strong magnetic field and a Feshbach resonance. As a result entirely different regimes of superfluidity can be accessed and investigated.
If the fermions slightly attract each other, Cooper pairs of two fermions are formed in close similarity to pairs of electrons in metallic superconductors like Niobium or Mercury. The theory which successfully describes this regime is the well established BCS theory named after the three scientists John Bardeen, Leon Cooper, and John Schrieffer. It is possible to change the interaction such that always two fermions form a closely bound dimer or molecule which in itself is bosonic. Those composite bosons form a Bose-Einstein condensate (BEC) which is superfluid as well and can be described by the Bogoliubov theory.
The most fascinating regime is located between the BEC and the BCS superfluid states. The gas is dominated by strong interactions and it is here, where superfluidity is the most stable. Despite tremendous research efforts, so far no comprehensive theory could be developed to describe this complicated and strongly correlated state of matter. It is the understanding of exactly this strongly interacting state which might hold the key to design new superconducting materials with high critical temperatures.
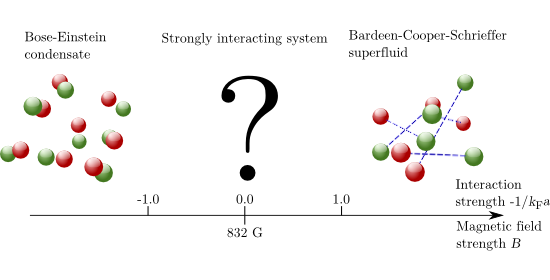
Critical velocity
One of the properties to quantify the stability of a superfluid is the critical velocity. If a massive obstacle is dragged through the medium it does not experience a drag force, as long as the movement is slow. However, if the velocity exceeds the critical velocity excitations are created which ultimately lead to heating of the superfluid and a drag force acting on the moving obstacle.
The elementary excitations which are created depend on the interaction regime the superfluid is in. In a BCS superfluid it is the breaking of the Cooper pairs which defines the critical velocity whereas in a BEC it is the creation of phonons. In the latter the critical velocity is therefore predicted to equal the speed of sound. In the strongly correlated regime the nature of the excitations limiting the critical velocity is unknown and experimental measurements are urgently needed to provide valuable information.
Experiment
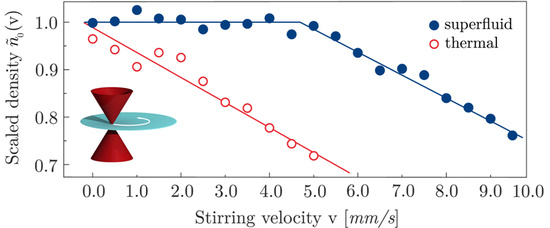
Our team working on the lithium experiment performed such a measurement. The corresponding publication can be found here. A highly focused laser beam, serving as moving obstacle, was guided onto a flat cloud of ultracold lithium. The beam was moved through the cloud on a circular trajectory to measure the minimal velocity needed to cause heating. Depending on the interactions strength different critical velocities were observed which were highest close to the point where the interactions are the strongest. We found, that even in the BEC regime the established Bogoliubov theory is not able to predict the observed critical velocity correctly.
Extensive simulations performed in the group of Ludwig Mathey were able to reproduce and explain the effects which lead to the reduction of the critical velocity in the BEC regime.
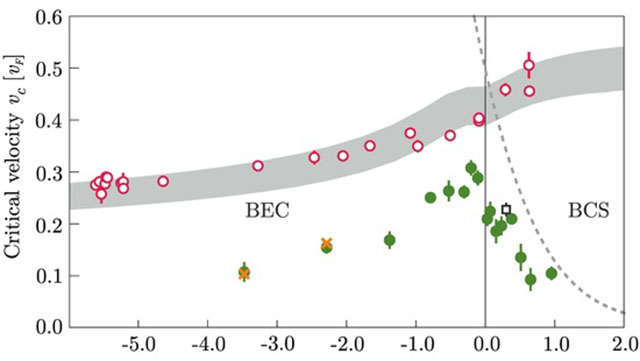
Towards lower dimensions
The situation we created in the experiment is closely related to the flow of Cooper pairs in a superconductor around a point like defect.
Of particular relevance in modern technology are superconductors with high critical temperatures. Their superconducting properties are known to be linked to strong interactions and the presence of two-dimensional planes within the crystal structure. The reasons behind are unknown.
The lithium experiment in our research group is able to create strongly interacting two-dimensional layers of ultracold fermions and experiments on those gases might be able to provide insights into the underlying physics of high temperature superconductivity.