Research Area B
Control and study of strong correlations and quantum phases in condensed matter
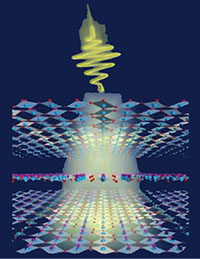
Understanding the vast variety of phases that exist in solids even of remarkably simple structure and chemical composition has been a major topic in condensed matter physics over the last century. Ultra-short laser pulses have opened an entirely new direction in this field of research, by allowing the study of condensed matter systems on time scales on which their microscopic constituents are not even locally in thermal equilibrium. These studies come with several great promises and goals, which are among the overall goals of this SFB: On the one hand, looking at how the relaxation of exited states proceeds in time can help to unravel the origin for the complex phases in equilibrium. Most importantly, however, ultrafast pump-probe experiments evoke the dream of a new era in material science in which properties are controlled at ultrahigh speeds by rapid stimulation (e.g. by light). This may also lead to new concepts for micro and nano-technological applications, e. g. devices, which allow switching within femtoseconds, magnetic storage which is controlled all-optically on the time scale of the exchange interaction, or a better understanding of transport and diffusion processes in strong potential gradients, which can help to improve the efficiency of photovoltaic devices.
In all these examples the class of materials, generally referred to as strongly correlated electron systems, promise important advances. In these systems, strong interactions between microscopic degrees of freedom emerge from very high-energy scales, which make the resulting phases robust against violent thermal fluctuations and allow for macroscopic quantum phenomena at very high temperatures. The emergence of superconductivity above 100 K is a spectacular example of such robust macroscopic behavior. Nevertheless, these phases can often be accessed and changed by relatively weak perturbations, as a result of the highly nonlinear effects arising from the collective behavior of many particles. These properties make correlated materials unique also for the study out of equilibrium: The emergent nature of interactions suggest possibilities to use light with frequencies corresponding to several electron-volts to design effective forces acting selectively and very strongly on the microscopic degrees of freedom. Moreover, the variety of phases that arise from collective behavior in equilibrium can be expected to be complemented by an equally bewildering variety of metastable phases that exist only under non-equilibrium conditions and can be accessed to achieve material control on femtosecond time scales.
Many conceptual hurdles stand in our way, largely because we have no effective way of predicting the behaviour of complex solids with accuracy. Our goal is to make conceptual progress towards a concise understanding of non-equilibrium processes, a feat that requires frontier instrumentation and a new generation of light sources, as well as frontier theoretical techniques. Research area B uniquely combines the ability to control and probe non-equilibrium states of strongly correlated materials with light sources that span several orders of magnitude in photon energy, from phase-stable THz sources to X-rays from free-electron lasers.
Individual Projects
Project | Title | Principal Investigators |
---|---|---|
B2 | Ultrafast dynamics in strongly correlated systems | Rossnagel |
B3 | Correlations and symmetries in spin systems | Grübel |
B5 | Dynamics of strongly correlated degrees of freedom in model systems | Potthoff |
B7 | Towards metastable non-equilibrium superconductivity | Cavalleri, Mathey |
B8 | Femtosecond science on-chip: Controlling non-equilibrium quantum transport in graphene heterostructures | McIver, Cavalleri, Mathey |
B9 | Controlled dynamics in crafted spin arrays coupled to itinerant electron baths | Wiebe, Krause, Wiesendanger |