Nonlinear photoionization reveals hidden properties of collective excitation
1 June 2015
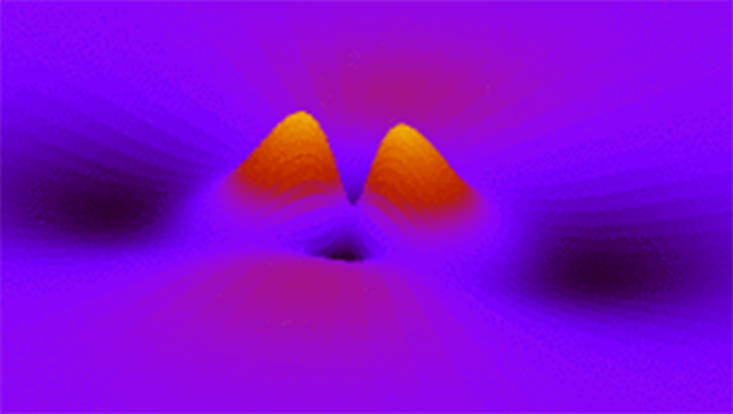
Photo: Antonia Karamatskou, I. Institute of Theoretical Physics, Project A5
Combining photoionization with photoelectron spectroscopy has proven to be a successful tool for the investigation of electron dynamics and many-body interactions. Our joint experimental and theoretical analysis shows for xenon as a model system how nonlinear XUV spectroscopy reveals features in the collective electronic response that remain hidden in linear spectra. The results support the prediction of two distinct resonances underlying the xenon 4d giant dipole resonance.
Xenon is a prime example of an atomic system exhibiting electron correlation effects. Its strong response to XUV radiation, the giant dipole resonance (GDR), was discovered in the 1-photon absorption spectrum [1]. It is interpreted as the interplay of two effects: A photon excites an electron from the 4d shell to the continuum where it is temporarily trapped until it tunnels out and leaves the ion. However, quantitative agreement with experiment is only achieved if correlation effects within the 4d shell are included [2]. This means that the broad resonance is a result of the collective response of all electrons in the 4d shell. The 4d0 orbital is depicted graphically in Fig.1. So far, the GDR was viewed as a single, broad resonance associated with a single quantum state. We showed that XUV 2-photon spectroscopy is sensitive to resonance substructure which remains unresolved in 1-photon spectroscopy.
Today free-electron lasers enable the investigation of collective excitations beyond the linear response regime. To study the nonlinear 4d ionization of xenon we employed photoelectron spectroscopy in combination with first-principles calculations. The experiment was performed at FLASH at 105 and 140 eV photon energy which lie in the range of the GDR. Pulses of various intensities were focused to a few microns and the photoelectrons were collected with a magnetic bottle spectrometer. The resulting photoelectron spectra contain the 1- and 2-photon absorption lines separated by the energy of one photon. Our theoretical model includes many-body effects and allows for the selective inclusion of correlation effects [3]. The cross sections for 1- and 2-photon ionization are calculated both including correlations and switching them off (Fig. 2b and 2a) in order to relate the effect to the collective response of the system. The corresponding electron yields are obtained via rate equations and c ompared with the data (top panel Fig. 2).
Fig.1: Graphical representation of a 4d0 electron orbital in atomic xenon. In order to describe the 4d giant dipole resonance correctly all 4d orbitals have to be taken into account.
Excellent agreement is obtained at both photon energies when accounting for a collective response, i.e., including the Coulomb coupling between all possible particle-hole states. The single-particle model fails to reproduce the data. This validates our theoretical model and the importance of correlations also in the nonlinear regime.
Analyzing the 2-photon cross section over the full range of the GDR we find that, surprisingly, the 2-photon cross section is broader than the 1-photon cross section and exhibits a knee-type structure. This shape cannot be explained if only a single intermediate resonance state is assumed (blue curves Fig. 2), because in this case the cross section could be described as the product of two cross sections for the two ionization steps which results in a narrower profile. Instead, if a second resonance state is assumed the 2-photon cross section is characterized by the occurrence of interference terms between the two intermediate states. This results in the broadening and the knee structure (red curves Fig. 2a,b).
Fig.2: Top panel: Comparison of experimental (full points) and theoretical (lines) electron yields as a function of intensity at 105 and 140 eV. Solid lines: electron correlation effects (CE) included. Dashed lines: correlation effects switched off. Panel a) Model without CE, panel b) Model with CE. The blue curves assume a single intermediate resonance state. The red curve in panel b) is the two-photon cross section including CE, which reproduces the experimental results at both photon energies. Being broader than the one-photon cross section (black curve) it exhibits a knee-type structure hinting at the second resonance state underlying the 4d GDR.
We conclude that the agreement of experiment and theory in the nonlinear response regime legitimizes the prediction of two quantum resonance states underlying the xenon GDR. Nonlinear XUV spectroscopy provides significant sensitivity to unresolved substructure in the collective excitation of many-body systems.
References
1. D.L. Ederer, Phys. Rev. Lett. 13, 760-762 (1964); A.P. Lukirskii, I.A. Brytov and T.M. Zimkina, Opt. Spectrosc. (USSR) 17, 234-237 (1964).
2. M. Ya. Amusia and J.-P. Connerade, Rep. Prog. Phys. 63, 41-70 (2000).
3. L. Greenman et al., Phys. Rev. A 82, 023406 (2010).
Antonia Karamatskou, I. Institute of Theoretical Physics, Project A5
Original publication
“Sensitivity of nonlinear photoionization to resonance substructure in collective excitation”
Nature Communications 6, 6799 (2015). DOI: 10.1038/ncomms7799
Authors: T. Mazza, A. Karamatskou, M. Ilchen, S. Bakhtiarzadeh, A. J. Rafipoor, P. O’Keeffe,
M. Kelly, N. Walsh, J. T. Costello, M. Meyer and R. Santra