Understanding superfluidity via laser stirring
1 December 2015
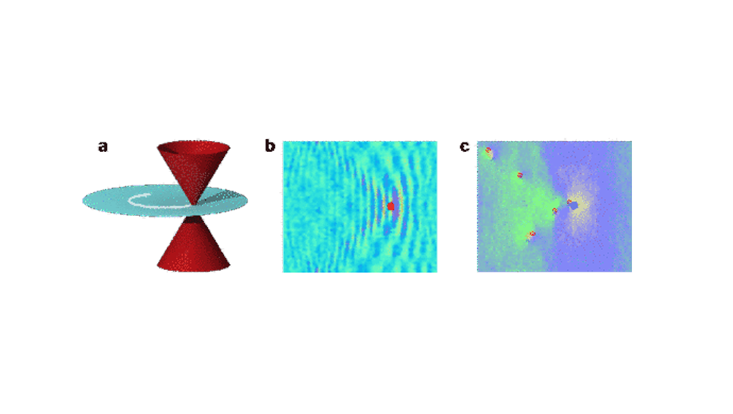
Photo: Vijay Singh, Centre for Optical Quantum Technologies, Project C9
Superfluids have the surprising property that obstacles can move freely in them without experiencing friction. In contrast, when pulling a stick through water, one clearly feels a resistance. This intriguing phenomenon of superfluidity was first observed in liquid helium 4 and helium 3. With the technological advance of atomic traps and laser cooling, the well-controlled and tunable experimental platform of ultracold atom systems has emerged, which allows further study of this phenomenon and its many intriguing features.
In these experiments, atoms are cooled to a millionth of a degree above absolute zero. They undergo a phase transition to a superfluid state which is then studied by dragging a laser beam through it. As predicted by the Landau criterion, this should result in no measurable heating for velocities of the laser beam below a threshold velocity, which is referred to as the critical velocity. In the group of Henning Moritz (project C4) at the ILP, the superfluid behavior of a Bose-Einstein condensate (BEC) of 6Li molecules was probed in this manner. A schematic diagram of the experiment is depicted in Fig. 1a: a circularly moving, red-detuned laser beam stirs the condensate to measure the critical velocity. In contrast to superfluid helium, here the interaction strength between the fermionic atoms can be tuned freely, allowing to measure the critical velocity in the crossover from Bose-Einstein condensation to Bardeen-Cooper-Schrieffer pairing.
Fig. 1. a: Schematic representation of the stirring experiment performed in the Moritz group (project C4). A circularly moving, red-detuned laser stirs the condensate, depicted as a circular disk. b: Computer simulation of a red-detuned laser that is dragged through a condensate. It dissipates energy due to the creation of phonons. c: Dissipation also occurs via vortex-antivortex creation (circled-dot and cross) if the laser is blue-detuned.
The work was performed in close collaboration within the SFB with the theory group of Ludwig Mathey (project C9). It provides a quantitative understanding of the superfluid response of the molecular BEC. Using both analytical and simulation methods, a theoretical prediction for the critical velocity of the BEC was determined, and compared with the measured critical velocity. The comparison to the experiment provides a systematic insight into superfluidity. Firstly, it identifies the dominant dissipation mechanism under different experimental conditions. For a red-detuned laser, which results in an attractive stirring potential, superfluidity decays due to the creation of phonons (Fig. 1b), whereas for a blue-detuned laser, and therefore a repulsive stirring potential, it decays via the creation of vortex-antivortex pairs (Fig. 1c). Furthermore, to achieve a quantitative understanding, the temperature of the system has to be taken into account, as well as the geometry of the stirring path.
This joint study extends the understanding of superfluidity by identifying some of the key features that influence the dissipative processes of superfluids. These insights guide the continued investigation of superfluidity, such as superfluidity in fermionic systems and dissipation in strongly correlated states, as well as the study of related phenomena such as superconductivity.
Vijay Singh, Centre for Optical Quantum Technologies, Project C9
Original publication
Original publication: “Probing superfluidity of Bose-Einstein condensates via laser stirring”Physical Review A 93, 023634 (2016)Doi: 10.1103/PhysRevA.93.023634Vijay Pal Singh, Wolf Weimer, Kai Morgener, Jonas Siegl, Klaus Hueck, Niclas Luick, Henning Moritz, and Ludwig Matheyand“Critical Velocity in the BEC-BCS Crossover”Physical Review Letters 114, 095301 (2015)Doi: 10.1103/PhysRevLett.114.095301Wolf Weimer, Kai Morgener, Vijay Pal Singh, Jonas Siegl, Klaus Hueck, Niclas Luick, Ludwig Mathey, and Henning Moritzck, Ludwig Mathey, and Henning Moritz